Spanning Space
Plumbing the Depths of the Cosmos
When you read of the distance to the Moon, or Mars or a remote galaxy, have you ever wondered how these distances were measured? You can't do it with a ruler or tape line or pace it off so other methods must be used.
One of the most basic techniques for distance measurement is called triangulation. Knowing the length of one side of a triangle (the base-line) and of two angles you can determine the lengths of the other two sides. Special instruments are commonly used to measure the angles but even without them a person continually determines distances by using both eyes as a triangulation system, the distance between the eyes being the base-line.
Triangulation with two eyes works well for judging the distances of nearby objects but for determining the distances of remote objects larger base-lines are needed. The larger the base-line the larger the distance that can be measured. Indeed, with a big enough base-line the distance to astronomical objects can be determined.
For example, with an intercontinental base-line, the distances to the moon and planets have been measured. However, even with the earth's full diameter for the base-line, the method is inadequate for measuring the distance of even the nearest star (4 light-years) because the angles become so nearly 90° that even the most precise instruments won't do.
With the advent of RADAR (an acronym for Radio Direction And Range) another method of distance measurement involving time delays became available following W.W.II. With radar a pulse of radio waves is sent out and the time for the echo to return from an object is measured. Multiplying the echo time by the wave velocity gives the distance. 1 [1. In symbols, R=vt/2, where R is the distance (in kilometers), v is the velocity of the radio wave (equal to 300,000 kilometers per second) and t is the delay time for the echo (in seconds).] This method is so accurate that the distance from the earth to a point on the Moon can be measured with a precision of a few centimeters. The distances to some of the inner planets of the solar system have also been measured by radar but the long time delays (hours) and weak echoes make radar marginal for the remote planets. However, with radio-equipped probes, such as Voyager 1 and 2, the method can reach further since when the probe "hears" the pulse sent from the earth it can send back an amplified echo.
To stretch triangulation beyond the solar system, the diameter of the earth's orbit has been used as a base-line. With this base-line the distances to stars have been measured out to about 300 light-years. 2 [2. A light-year is the distance light travels in a year. It is equal to 10 million billion meters (1016 meters).]
For still greater distances the fundamental fact that the brightness of a star decreases as the square of the distance has been applied. Thus, at twice the distance, a star will be one-quarter as bright. So if we know how many watts a star radiates then a measurement of its brightness can tell us how far away it is. 3 [3. In symbols, R=√(W/4πB), where R is the distance (in meters), W is the power or wattage (in watts) and B is the brightness (in watts per square meter). √ is the square root operator.]
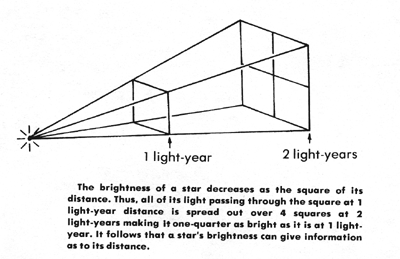
Stars have been grouped by their spectra 4 (and other means) into different classes and the wattage levels for the classes determined, at least approximately, by triangulation measurements of some of the nearer members of each class. The distances of other members of a class, too distant for triangulation, can then be inferred from their brightness. [4. The light from a star passed through a prism produces a spectrum or rainbow of colors and lines indicating the temperature and chemical composition of the star. See COSMIC SEARCH, Summer 1970 (Serial No. 3) page 37 and Winter 1981 (Serial No. 9) Page. 4.]
A particular star class that has proven especially useful for distance determinations is the Cephied variable [sic; "Cephied" should be "Cepheid"] group. These stars dim or brighten cyclicly with periods of days or weeks.
We can't span space with a ruler but mankind has developed an impressive kit of tools which can.
About 1908, in studying some of these stars in the Magellanic clouds (small nearby galaxies), Henrietta Leavitt of Harvard University noted that the brighter ones pulsed more slowly than the fainter ones. Since all were at roughly the same distance this meant that the slowly varying Cephieds [sic; "Cepheid"] were intrinsically more powerful than the more rapidly varying ones. A determination of the wattage of one of the Cephieds [sic; "Cepheid"] then served to calibrate all of them as standard wattage stars so that the (average) wattage of any of them is known if its period is known. Thus, a slowly varying Cephied [sic; "Cepheid"] variable which appears faint must be far away like a powerful lighthouse at a great distance.
Subsequently, Harlow Shapley of Harvard used the brightness of Cephied [sic; "Cepheid"] variable stars in our own galaxy to establish our galaxy's diameter as 100,000 light-years with our solar system over half-way to the edge. 5 [5. See COSMIC SEARCH, Winter 1981 (Serial No. 9) page 20.]
Then in the 1920s, Edwin Hubble, a lawyer turned astronomer, used the Mt. Wilson 2.5-meter telescope, the largest then in existence, to resolve some of the nearer external galaxies into stars and among then he found Cephied [sic; "Cepheid"] variables. Using these he established the distance of some of these galaxies at a million or more light-years.
Hubble with Milton Humason, his observing assistant, also photographed spectra of these galaxies. They noted that the light from the galaxies was shifted to longer wavelengths by an amount which increased with the distance of the galaxy. This shift to longer or redder wavelengths, called a redshift, implied that the galaxies were receding from us with a velocity which increased in proportion to their distance and this led Hubble to conclude that we live in an expanding universe. 6 [6. The redshift is thought to be produced by the Doppler effect, a phenomenon which we often experience audibly as the decrease in pitch (increase in wavelength) of the horn of a truck or locomotive that is moving away from us. See COSMIC SEARCH, Fall 1979, (Serial No. 4) page 25.]
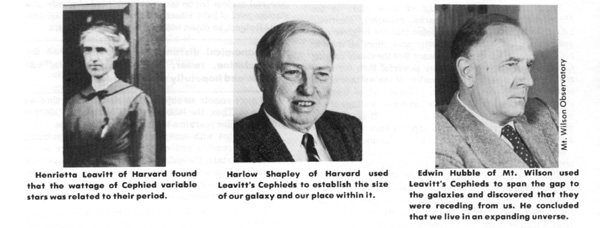
Going backwards in time, like running a movie film of the universe in reverse, the expansion appears to have started about 15 billion years ago with a titanic explosion called the Big Bang. Assuming that all objects in the universe partake of the expansion, a measurement of any object's redshift can tell us how far it is. 7 [7. The redshift gives the velocity and the velocity the distance. In symbols, R=vT, where R is the distance (in meters), v is the velocity (in meters per second) and T is the age of the universe (in seconds). See COSMIC SEARCH, Spring 1980 (Serial No. 6) page 32 and Summer, 1980 (Serial No. 7) page 22; also OUR COSMIC UNIVERSE by John Kraus, Cygnus-Quasar Books, 1980, Pages 59 and 182.] In this manner, distances have been deduced for quasars almost to the "edge" of the universe. 8 [8. The term "edge of the universe" is metaphorical. More properly it is a celestial horizon or observational limit.] The most distant known object is quasar OQ172 at 91 percent of the way to the "edge". The most distant galaxies Hubble measured are only a few percent of the way and the most distant known galaxies are about 60 percent of the way. A galaxy is a huge aggregation of 100s of billions of stars. Many astronomers believe that a quasar is a galaxy with an extra-powerful nucleus but so far away that it appears only as a starlike point of light. Hence, the name QUASAR for QUASi-stellAR object, an object which looks like a star but isn't.
Astronomical distances can be measured by triangulation, radar, brightness, redshift, expansion and hopefully soon by radep.
The more remote an object, the farther back in time we observe it. Thus, the look-back time for quasar OQ172 is almost 14 billion years in a 15 billion-year-old universe.
So it is that with many steps involving triangulation, brightness, redshift and expansion, the distances to the planets, the stars, the galaxies and the quasars have been spanned and measured. And now comes a new technique, RADEP for RAdio DEPth, which gives promise for measuring all astronomical distances with one technique.
Although radep is a form of triangulation, it works on the principle that the response pattern of a radio telescope antenna can be affected by the distance of the radio source being observed. Thus, if the distance of the source to the edges of the telescope differs by a fraction of a wavelength from the center of the telescope the pattern is different. When a point source is at more than a certain critical distance, the pattern is always the same. At less than this critical distance the pattern is not only different but changes with distance. 9 [9. In the esoteric language of antenna designers, it is said that beyond the critical distance the source is in the far-field or Fraunhofer region of the telescope antenna while inside the critical distance it is in the near-field or Fresnel region. For a one-eighth wavelength path difference we have in symbols, R=L2/λ, where R is the critical distance, L is the transverse size of the telescope and λ is the wavelength (all dimensions in same units). See, for example, ANTENNAS by John Kraus, McGraw-Hill, 1950, pages 117 and 448.]
The farther away an object, the farther back in time we observe it. With quasar 00172, we are looking back almost 14 billion years into the history of our 15 billion-year-old universe.
For radep measurements of astronomical distances, the radio telescope must be in space in order to avoid atmospheric effects and to permit building a telescope of sufficient size. A group of Soviet scientists, including Nikolai Kardashev and Josef Shklovsky, have proposed an interferometer radep telescope of three units, two deployed near the orbit of Saturn with the third near the earth. 10 [10. Report PR-373, Academy of Sciences USSR, Space Research Institute, Moscow, 1977.
Note: Other characteristics of objects, such as size, can also be used to gauge distance. Thus, galaxies of a given class which appear small should, on the average, be farther than those which appear larger. Although size might also be used for judging the distance of stars of a given class, it is not generally feasible to do this because, except for a few of the nearest largest stars, their diameter is not measurable, their images appearing only as points of light.]
Operating at centimeter wavelengths, this radep array has the potential, in principle, of measuring the distance of celestial objects all the way to the "edge" of the universe. A new astronomical era will be opened when radep can be employed to determine the distances of the quasars to see if they agree with those now quoted on the basis of our present multi-step procedure involving triangulation, brightness, redshift and expansion.
Although we can't span space with rulers or tape lines, mankind over the years has developed an impressive kit of tools which can.
ASTRONOMICAL DISTANCE MEASURING TECHNIQUES |
To: | Techniques: |
Moon, Sun and planets of solar system | Triangulation with base-line on the earth. Radar. |
Nearest stars | Triangulation using diameter of earth's orbit as base-line. Star brightness. Radep. |
More distant stars | Star brightness. Radep. |
Nearest galaxies | Star brightness. Galaxy size. Radep. |
More distant galaxies and quasars | Galaxy size and brightness. Red shift and expansion. Radep. |