![[NAAPO Logo]](../../Images/NAAPOsm.jpg)
North American AstroPhysical Observatory (NAAPO)
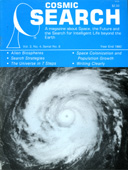
Cosmic Search: Issue 8 (Volume 2 Number 4; Year End 1980) [All Articles & Miscellaneous Items]
|
Webpage Table of Contents (Bookmarks)
(Internal links to items in this webpage)
[Note. Use Back button (or <Alt>+<Left Arrow>) to get back to this Table of Contents after you have clicked on a link and viewed the article.]
Codes Used Below:
P: Starting page of article in magazine; A: Author(s); T: Title of article
The Recognition of Alien Biospheres
By: James E. Lovelock
Introduction
The captain of a spacecraft approaching a new planetary system calls his exobiology officer and asks him to determine whether any of the planets bear life. With a remote sensing device the officer is soon able to provide a confident yes or no answer.
Although this is a familiar scenario of science fiction, is such a device really feasible? Let us consider the possibilities.
I have previously suggested that the chemical composition of a planet's atmosphere could be used as an indicator of the presence or absence of life using observations with a telescope from afar.* (* Nature, 1965, vol. 207, p. 568; Icarus, 1967, vol. 7, p. 149.) If the biology on a planet, such as the Earth, merely adapted itself to the chemical composition of its atmosphere without changing it, then the idea would not work. However, if the atmosphere is actively affected and altered by life on the planet, then its composition could provide definite clues about the presence of life. If fact [sic(?); should "If fact" be "In fact"?], with Lynn Margulis, I have gone further and proposed an hypothesis that the Earth's biosphere (atmosphere, oceans, and crust plus plant and animal life) is a system which is able to adjust the environment to be an optimum for its needs. In this, the Gaia hypothesis, this optimization applies not only to chemical abundances in the atmosphere but also to the climate, the surface acidity and other important planetary properties.
Defining the Problem
The problem lies not so much in the design of the telescope as in the search procedure and in the interpretation of the information it gathers. We are so accustomed to recognizing terrestrial life by instinctive processes, that the basis of this recognition is rarely analyzed or questioned. To recognize alien life at a distance through a telescope we need something more than our internal terrestrial life recognition program. The science of heat transfer (thermodynamics) provides clues and guidance.
First, there is a requirement known as the second law of thermodynamics that systems run irreversibly "downhill" toward a state of equilibrium. Thus, mixing a glass of hot water with a glass of cold water results in a warm water mixture. This process is irreversible in the sense that the warm water mixture cannot be divided again into a glass of hot water and one of cold. However, life processes can go against this irreversible downhill trend by taking in substances or free energy from the environment.
If the life of a planet is chemically based and driven by a star's radiation, gaseous products may diffuse into the atmosphere and by their presence cause a departure from the equilibrium state. Thus, the atmosphere becomes a part of the system.
Second, it is intuitive to link life as a system with those processes which occur when the change of free energy is larger than a certain value. Such a limit is well recognized in studies of fluids as the Reynolds number which marks the onset of vorticity or whirlpool-like formations. Like life, vortices establish characteristic recognizable forms with life-spans and histories, and when they form they confer a new sort of dynamic stability upon their environment. On the larger scale in the atmosphere, such vorticity sometimes evolves to the intricate structure of a hurricane and a recognizability great enough to qualify for personal naming.
The importance of this development for the problem of life detection is that it provides a formal distinction between irreversible processes and life-like processes.
For example, within the appropriate range of something equivalent to a Reynolds number a planet might be expected to bear life whereas outside that range it would not. Maelstroms do not develop in duck ponds, neither could life start or continue in an environment which was otherwise ideal, but which lacked a free energy rate of change of sufficient magnitude. Such may well be the conditions deep on Jupiter, warm, damp, with all of the chemical components but lacking free energy. The possibility that convection may convey sufficient reactive molecular species from the upper atmosphere leaves open the question of the possibility of life on Jupiter.
Third, from the standpoint of probability, living systems possess a molecular configuration of extreme improbability when viewed against the highly probable equilibrium background condition.
Designing the Experiment
Turning now to the experiment, let us assume that the planet can be seen clearly enough through the telescope to provide the following information:
(1) Surface temperatures to within ± 20 degrees (kelvin).
(2) The presence of bulk water and ice and its surface distribution.
(3) The atmospheric abundance of chemically active gases down to one part in 100 million.
(4) The distribution of temperature, pressure and chemical composition with altitude and with surface coordinates.
The resolution of this last measurement should be sufficient to distinguish between at least the major mixing zones of the planet. For the Earth this would include the northern and southern hemispheres, the troposphere, the stratosphere and the upper atmospheric regions.
The methods are already at hand to gather most of these quantities for a planet such as Mars from the distance of the Earth. The instrumental methods would be spectroscopic and range in wavelength from the short ultra-violet to the long infra-red. To gather the information requires no visionary instrumental development, merely some improvements in current hardware.
This approach to life detection assumes that the life is chemically based, that it is situated at the planetary surface and that it uses the atmosphere as a transport medium and also as a storage space for raw materials. Even if it is possible to distinguish any departure from equilibrium associated with life, it can only apply if the life in question has modified that part of the planet which is observable. If life was too sparce ["sparce" is spelled "sparse" in the U.S.] or existed only below the surface of the planet, neither it nor its effects might be visible. There are reasons for believing, however, that once life is initiated on a planet it can only persist if it is able to control the planetary environment. This ability may be an important evolutionary step in the early stages of life. The early growth of life on a planet will certainly change the surface and atmospheric composition and such changes could easily alter the radiation environment to a state unfavorable to life. A failure to learn to control the planetary conditions at this early stage could be fatal. Life as a going concern is likely to be intense and to have profoundly modified the physical and chemical environment of the planet.
In developing the Gaia hypothesis, Margulis and I postulated that the biosphere has properties which enable it to control at least the following variables:
(1) Surface temperatures.
(2) Atmospheric composition.
(3) Surface and ocean acidity.
(4) Ocean composition.
(5) Materials balance.
In this hypothesis the biosphere is, like its constituent plant and animal parts, seen as a complex automatic control system with the capacity to adjust its environmental balance to its advantage. At present Gaia is only a hypothesis but if we can establish the reality of a tendency toward stability for the Earth then it may also be a general characteristic of biospheres. If it is, their recognition from afar will be much easier.
Experimental Evidence
Now let us consider the information available about the planets Jupiter, Mars, Earth and Venus in this context. To keep within the constraints of a realistic experiment the information about the Earth is restricted to that which could be gathered from the distance of Mars.
The figure shows the amount of chemically reactive gases in the atmospheres of the four planets; also included are the gases of a lifeless or sterile Earth. The gas concentrations are expressed in logarithmic units so that the minor constituents can be compared as well as the principal gases.
The outstanding difference between the (actual) Earth and the other planets is the simultaneous presence of molecular hydrogen, methane and ammonia with that of oxygen. If the gases of the atmosphere were allowed to react among themselves and with the crustal rocks and ocean the final equilibrium would be that marked Sterile Earth. One indication of life is the presence of these gases (hydrogen, methane and ammonia) in the actual Earth's atmosphere in amounts which are more than a trillion trillion (1024) times greater than they would be in a sterile Earth's atmosphere.
When considered singly and in isolation, plausible inorganic origins can be assigned to most of these gases but when the ensemble is considered together with oxygen we have something so unusual as to be beyond any credible inorganic chemistry. Any lingering doubts are dispelled by a consideration of the quantities of these gases. To sustain the present concentration of methane requires a billion tons a year and, also equally significant, 4 billion tons a year of oxygen. This much methane would require that all of the carbon in Earth's crust was turned over every 100 million years. This is normal for a biosphere but incredible as an accident. The discovery of almost any biochemical in neutral or reducing environment, even a protein, would not be conclusive evidence of life. The discovery of even a simple reduced carbon compound such as methane in sufficient quantity in an oxydizing atmosphere is, however, strong evidence for a dynamic system which is making it.
Atmospheric Recycling
The atmosphere is a huge mixing machine. It contains about 6 million billion (6 x 1015) tons of air. The earth's human population breathes about 25 billion tons of air per year so that humans breathe 4 parts per million of the atmosphere per year. Put another way, this means that for each breath a person takes, 4 parts per million were breathed by some human during the previous year. This amount could be more or less if mixing is incomplete. This is a measure of human air recycling which will increase in proportion to the population.
There are about 20 billion trillion (20 x 1021) atoms in a breath of air. Thus, for each breath one takes there are more than a million billion (1015) atoms in that breath which were exhaled by other humans during the past year and almost a billion atoms that were breathed by
any adult human that ever lived.
— Ed.
|
Other Biospheres
Even though the discovery of life on the Earth through the recognition of its biosphere may not be difficult, it does not follow that this is a generality. We cannot yet test the method against other planetary biospheres, but we can ask if it could have detected past biospheres on the Earth. We know that life has existed on the Earth for 3 billion years and although the view is poor from this distance in time some of the larger features can be seen. When life began there was probably more hydrogen and methane with less oxygen while during the evolution of the Earth's biosphere there has been a shift in the amounts.
If we assume that soon after the start of life a biosphere with automatic control is established; that the planet is developed to the limit of its resources and of the energy it receives from its star; that it is maintained at optimum conditions for whatever is the current biosphere; then the
amounts of these gases indicate the status of life processes on the planet as viewed from afar.
Truly Alien Biospheres
What of truly alien biospheres? The universe appears to be littered with the spare parts from which our current chemical life form is composed. From this fact and from our knowledge of properties of the elements it seems unlikely that other, for example, silicon based life forms are likely to have evolved. On the other hand, we have ample experience with the possibility of life based on electronic and mechanical contrivances; a biosphere in this form would evolve presumably from a chemical one, perhaps starting in cooperation with it. Could we recognize such a biosphere? If it is a property of biospheres to optimize their use of raw material and free energy to control the planetary surface conditions at those most favorable for survival, then this form of biosphere or an alien chemical one should be recognizable. Except by a purposeful act of camouflage any life system will reveal its presence through the chemical disequilibria caused by its contrivances.
Compounds now present in the Earth's atmosphere which might be seen by infra-red analysis from afar are the chloro-fluoro-carbons — the highly contrived chemicals made for use as refrigerants and aerosol propellants. They could not have arisen accidentally by inorganic chemistry and their presence would be indicative, for example, of a biological life which had developed a chemical industry.
Much of this discussion has been concerned with the properties of planetary atmospheres. This is partly because more information is likely to be available about planetary atmospheres than about their surfaces and partly because the physical chemistry of low pressure gas mixture is considerably easier to consider than mixtures including also solids and liquids. But a view of a planet does provide information on such surface features as clouds, oceans, reflectivity, radiation and the distribution of temperatures and chemical substances. These all can add weight to the sum of evidence concerning the presence or absence of life.
Conclusions
This work started 15 years ago, motivated by the need to design a planetary life detection system which was not geocentric and could be used, for example, for Mars. Applied to the Earth, which is the only planet we know with life, there is a great difference in its atmospheric gases from those expected in the absence of life (sterile Earth). By contrast, what we know about the other planets shows no sign of a significant departure from that expected for a sterile biosphere.
If future planetary explorations confirm the absence of life on the other planets of our solar system, then the remote detection of life by telescope might be established as a method, at least for planets like the Earth. It may even be a general method of planetary life detection and the remote sensing device of science-fiction could become a reality.
It may be some time before we can try these tests for life on the planets of other stars but in the meanwhile what started as an exercise in life beyond the Earth could become an expedition to discover the largest living creature on Earth, Gaia.
This article is a popularized condensation based on "Thermodynamics and the Recognition of Alien Biospheres" by James E. Lovelock, Proceedings of the Royal Society (London), 1975, vol. 189, p. 167.
James E. Lovelock was born in Letchworth Garden City, United Kingdom, in 1919. He received his Bachelor's degree in Chemistry from Manchester University in 1941 and his Ph.D. degree in Medicine from the London School of Hygiene in 1948.
For nearly 20 years he was with the Medical Research Council both at Salisbury and London. In 1954 he was at the Harvard Medical School on a Rockefeller fellowship and later was a visiting scientist at Yale University Medical School. He was a professor at Baylor College of Medicine and the University of Houston, Texas, from 1961 to 1964. Since then he has been a free-lance scientist serving as a consultant to various companies and institutions, with a continuing connection as a visiting professor to the University of Reading.
Dr. Lovelock has published nearly 200 scientific papers in medicine, biology, gas chromatography (or analysis) and atmospheric science. He is a Fellow of the Royal Society and in 1975 was awarded the M.S. Tswett Chromatography Medal. He has received an honorary Doctor's degree from London University and his cooperation in the space program was honored by NASA with a Certificate of Recognition.
Dr. Lovelock's primary interest is in the life sciences, originally in medical research but more recently in the role of the Biosphere in maintaining the surface conditions of the Earth as an optimal habitat for Life. He has also designed and developed instruments to pursue this interest. His involvement in gas chromatography goes back to his collaboration in 1956 with A.T. James on gas analysis of fatty acids found in lipids from lipoproteins and blood cells. During this work, he
developed the argon ionization detector which proved to be the origin of a family of detectors. The most important member of this family was the electron capture detector which revolutionized environmental analysis.
|
Strategies of Searching for Extraterrestrial Intelligence
A Fundamental Approach to the Basic Problem
Part Two, continued from last issue (No. 7)
By: Nikolai S. Kardashev
The examples given in the first part of this article in the last issue of COSMIC SEARCH cover only a few of the possible trends in the activities of civilizations. All of these examples, however, seem to be directly related to the correct strategy of searching for civilizations, a subject we shall
now discuss.
Right and Wrong Search Strategies
Two concepts and two extreme strategies of search for extraterrestrial civilizations are now being
discussed in the scientific community:
(1) The Concept of "terrestrial chauvinism": Each civilization stabilizes or perishes at a level of development close to ours (see the conclusions and recommendations of the "Club of Rome" regarding strict limits to population and its activities.)
Search Strategy: Search for civilizations of our type, i.e. belonging to a planetary system, using communication techniques similar to ours: monochromatic signals from the nearest Sun-like stars, gradually extending the survey radius.
(2) The Evolutionary Concept: Civilizations can attain much higher evolutionary level than ours.
Search Strategy: Search for super-civilizations with more powerful transmitting systems and highly advanced engineering activity. There are two directions for such studies. First, study the character of the most powerful (and often most distant) known sources of radiation in the Universe. Only after we are certain that their functioning is natural, is it worthwhile to begin surveys of weaker objects. Second, search for new and powerful sources of radiation, mainly in the poorly investigated regions of the electromagnetic spectrum.
Therefore, it would be worthwhile to search for meaningful monochromatic or pulsed signals from the
center of the Galaxy, from nuclei of other galaxies and quasars, from other classes of astronomical objects (if there are grounds to relate them to the evolution of civilizations), to search for traces of astro-engineering activities in these objects, with maps made with a super-high resolution, and to investigate in detail any "peculiar" sources which may be discovered (for instance, very large structures might be discovered, some of which might move at nearly relativistic velocities).
An optimum wavelength range to be used in the search for artificial signals from the nucleus of our
Galaxy and from the nuclei of other galaxies and quasars differs radically from the range most favorable for contacts with the nearest stars. The former objects whose nature is still unknown, are characterized by an intense natural radio emission of synchrotron origin, covering the whole radio-frequency range. Furthermore, radio waves are heavily scattered in the clouds of plasma surrounding these objects. Both of these factors imply that the most favorable search range is probably at millimeter wavelengths, near the intensity maximum of the relic background, rather than near a 21 centimeter wavelength. It is of interest that if a civilization in a distant extragalactic object sends signals in this spectral region, then, despite changes in the background spectrum during the signal propagation, the signals will also appear to arrive near the maximum intensity of the primordial fireball background as seen by observers in our Galaxy. For observers in our Galaxy, the spectral region of the maximum intensity for the primordial background corresponds to a wavelength of 1.7 millimeters. An attempt should be made to discover radiation in this region carrying specific information as well as thermal radiation from large astro-engineering structures. Therefore, it would be extremely interesting to investigate the nature of the radio point source (of size smaller than that of the solar system) which is located in the very center of our Galaxy and which radiates at short waves, as well as the nature of several sources of infra-red radiation near the Galactic Center whose temperature is close to room temperature.
Is there a danger of losing a sense of sound judgement if actual, observed astrophysical phenomena are interpreted in this way? At present, it is expedient to keep in mind both possibilities when unknown objects are studied: a "natural" explanation of objects which are evolutionary in the lifeless Universe, and models of objects which might be called "cosmic miracles" but which might also be the results of the long evolution of intelligent life in the Universe. Both concepts may stimulate experiments to show which of the two is closer to the truth.
Finally, several appraisals are given of the possibility of detecting at our present technological level, extraterrestrial civilizations. Such calculations are very reliable because they are based on the well-known propagation conditions for electromagnetic signals in space. The temperature versus frequency chart [below] shows the frequency dependence of brightness temperature of the cosmic background radiation and the location of the two main SETI search ranges. The range near the famous radio line of hydrogen at 21 centimeters corresponds to the presumption of omnidirectional transmission, and is intended for searches for signals from the nearest planetary systems.
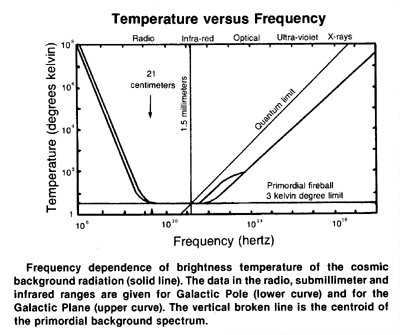
The range near the centroid of the relic background spectrum (wavelength about 1.5 millimeters) corresponds to the most economical strategy of space communication, namely, to align the transmitting and receiving antennas, and to send the radiation and the information it carries in a narrow beam. This is the optimum range for small as well as for large distances (the spreading of a beam of radiation is smaller in the millimeter range than it is near 21 centimeters). The millimeter range may also be used for the detection of large astro-engineering structures. For such a search, very high angular resolution is needed, which may be achieved only by radio with Very Long Baseline Interferometers (VLBI).
The first millimeter search for meaningful signals as well as for thermal emission or scattered radiation from astro-engineering structures may be conducted with existing and projected radio telescopes in both millimeter and centimeter ranges. But for positive results, it is probably necessary to have a special, very large radio telescope — an interferometer with a baseline much larger than the Earth's diameter. Such a large radio telescope must be constructed not on the Earth, but in space — in a geostationary, or more distant, orbit. Such an instrument would be able to completely reject the tremendous radio interference associated with the activity of terrestrial civilization. The estimated expenditure for such an instrument, with reflector sizes of several kilometers, may be even less than for a ground system with the same collecting area, because of the absence of gravity. The construction of such telescopes will be possible in the next decade. A project on space radio telescopes to be built with reflectors up to 10 kilometers in diameter was discussed by V.I. Bajakas, A.S. Gvamichava et al. in their paper "Infinitely Built-up Space Radio Telescope" delivered at the International Astronomical Union Congress in Prague in 1977. (See the SEnTInel in the January 1979 issue of COSMIC SEARCH). A similar project was proposed in the U.S.A. The natural radiation of astro-engineering structures, or their absorption or reflection of the background radiation or information-carrying signals of civilizations, could be detected at distances comparable with the curvature radius of the Universe with such radiotelescopes.
The graph of distance versus telescope size [below] illustrates these conclusions. Estimates are made for possible detection of astro-engineering structures of the Dyson-Sphere type at temperatures of 3 and 300 degrees kelvin radiating the same power as the sun does, but in the infrared and millimeter range, as well as for meaningful signals of about the same power sent by a supercivilization. The graph also shows detection capabilities for transmitters similar to those available on Earth, corresponding to the concept of "terrestrial chauvinism" (lower curves). Note that the range of detection, for the "evolutionary" concept (corresponding to the upper curves) is many orders of magnitude larger.
Conclusions
Astrophysical investigation as well as data provided by biology, cybernetics and other sciences show
that the detection of extraterrestrial civilizations is highly probable. All of the original concepts about what these civilizations may turn out to be and what search strategy should be followed must now be radically revised. In our opinion the only promising concept is the assumption that super-civilizations exist. The way to detect them is to conduct the following series of experiments:
First, search for and study blackbody-radiation sources whose maximum intensity falls in the millimeter and infrared ranges, with the aim of detecting very large structures. Such structures might also be detected by their screening effect or by the way they reflect the cosmic background radiation in various spectral regions. Very promising approaches might be synthesis maps of peculair sources by means of superlong-baseline interferometers, or the detection of very compact objects by scintillation (caused by the modulation of radiation passing through inhomogeneous plasma of space). Superhigh resolution in the interferometer regime and in scintillation observations requires radio telescopes separated by distances exceeding the Earth's diameter.
Second, search for meaningful signals from promising objects. The optimum wavelength range for such signals should be calculated, relative to the background radio emission near the respective source and the conditions of signal propagation in the plasma along the line of sight. The millimeter range is especially promising, near the maximum of the relic background radiation. These experiments might either reveal that some known astronomical objects are actually related to the activities of civilizations or else lead to the detection of entirely new classes of natural astrophysical sources.
If well-designed experiments based on the logically consistent Evolutionary Concept are carried out with future, largest-possible radio telescopes, the search for extraterrestrial civilizations could yield positive results within the first ten years, thereby giving mankind access to the vast store of information acquired in the Universe over a time span of billions of years.
I thank L. Gindylys, D. Downes, M. Popov, I.S. Shklovsky and V. Slysh for valuable discussions and assistance.
Dr. Nikolai S. Kardashev is a member of the Space Research Institute, Academy of Sciences, Moscow, U.S.S.R. His photograph appeared with the first installment of his article (in issue number 7). [However, I have reproduced that photograph here. — Wepbpage Editor] Dr. Kardashev is a member of the Editorial Board of COSMIC SEARCH.
|
SETI
By: F. (Frank) D. Drake
Detecting a SETI signal is in some ways the simplest of tasks, while in other ways it is the most difficult. A real signal has "intelligence" written all over it. But that signature can appear in countless guises; after all, we are surely dealing in SETI with a multitude of cultures far different than ours. Then what will be their electromagnetic hieroglyphs? Will we recognize them, especially if they flash only briefly through our electronic systems? Perhaps they will be ephemeral will-of-the-wisps — was it an intelligent signal which blinked past, a spasm of normal galactic radiation, or a signal from earth finding its way fleetingly into our receiving system? There is a profound problem here.
Perhaps I can explain better by describing an experience which happened a few weeks ago at Arecibo. Two graduate students, Chip Cohen and John Simonetti, and I were in the midst of a lengthy search for radio emission from a previously undetected molecule of particular interest, glycine. The most basic of amino acids, the stuff of life perhaps everywhere in the galaxy. To find it would encourage our beliefs that life is common in space. We were observing on a wavelength near 30-cm, a band not used in radio astronomy, and not protected from radio interference by the international agreements which protect most radio astronomy frequencies. Our observations were taking place in the middle of the night, and we were tired after many nights of this routine. We were seeing spectral lines, all right, perhaps from glycine but more probably from hydrogen. And we were impressed at the fact that we had received no radio interference at all in the many weeks of observing we had piled up in this frequency band. Actually, this is not too surprising because Puerto Rico is relatively isolated in the middle of an ocean, and most transmitters, even all the TV stations, turn off at night.
Every five minutes, the spectrum of the radio waves captured over the preceding five minutes would flash across the screen in the control room. Then, after hundreds of noisy repeats of the same spectrum, there suddenly appeared a spectrum with a new set of peaks in it: The complex yet obvious signature of an intelligent radio signal carrying information in the form of pulses. At 3:23 in the morning! And how eery: The signal was exactly on the radio frequency of the glycine molecule, that special place in the spectrum which we had been staring at vainly for weeks. What could be radiating such a signal, on such a frequency, at such a time?
Our telescope operator had noticed the sudden onset of a variation in the output of our receiver. It resembled the typical effect of a malfunction in the system which commands the beam of the telescope to track the slowly turning sky. But we quickly saw that in fact the signal was the cause, and that it was turning on and off at three second intervals. How odd, how striking, how exactly like our fondest speculation about the nature of the beacon signal of an advanced civilization! And then it was gone, too soon to conduct the classic test of moving the telescope beam to another part of the sky to see if the signal went away. If it disappeared it was cosmic, if not, it was of earth. In our concentration on the search for the makings of primitive life, we had not been ready for a sign of advanced life.
The next night we were ready. And sure enough, 3:26 a.m. the same three second blurting of pulses suddenly commenced. Again on the glycine frequency. Quickly the telescope beam was swept across the sky. The signal remained, just long enough to prove it was from earth and not from the stars. We have never seen it or any other signal in this frequency band again.
There are many lessons here. The difficulty caused by terrestrial transmissions is one. Another has to do with one of the most common SETI questions: "How would you know it is an intelligent signal?" That answer is "It is easy." Here the signal created some ten individual spectral lines, uniformly spaced in frequency, and having strengths relative to one another which matched in detail the special and complex mathematics of trains of pulses. The signal turned on and off, totally, with
very precise timing. There are many other ways the signal could have marked itself clearly as coming from "people."
The episode reminds us of important obstacles to success in SETI. What if the signal had been only about five times fainter? Although there is mathematics which could have clearly established its presence, we don't regularly apply such tests to our data. We might not have noticed the signal until days afterward, if at all. It would have been too late to test it for extraterrestrial origin.
More tantalizing, what if space is full of detectable ETI signals of brief duration, these signals falling on our planet like raindrops, one after another. Each one makes a brief, barely detectable splash, and then it is gone. Our present ways of observing, and even the sophisticated ones being implemented for use in the near future cannot cope with the situation. In none of our systems are we building in an automatic, quick reaction protocol to test possible transient SETI signals. I hope this is not a fundamental mistake.
The above episode is certainly not unique. It differed from the regular experience of radio observers only in the peculiarities and strength of a signal. My own observing experience, when being especially attentive for any receiver output which might be of intelligent origin, is that there seem to be weak signals just popping up out of the noise all the time. Responses at this or that frequency which seem to be more than the noise behavior of the receivers should cause. I often have the same feeling as when I am deep in a forest, and now and then sense faint, brief, vague reminders of the civilized world around me; the distant sound of a jet plane, an auto horn far away, the faint shout of a human. These whispers keep reminding me of the civilization which surrounds the forest. Could it be that in our radio observations we are hearing, all the time, the faint murmurs of countless other civilizations, blowing past the earth as on the wind? Or is it just one more example of the ability of the human mind to perceive patterns in randomicity where no real pattern exists?

Dr. Frank D. Drake is Director of the National Astronomy and Ionosphere Center of Cornell University which includes the Arecibo Observatory. Dr. Drake is a member of the Editorial Board of COSMIC SEARCH.
|
Why Is Scientific Writing Unintelligible?
By: Sharon Dunwoody
Among scientists, SETI researchers may be the ultimate communicators: they are trying to contact intelligent life beyond the earth, life that exists only in hypotheses and about which nothing is known.
To that end, SETI scientists painstakingly grope for symbols, sounds, wavelengths that may have meaning to life elsewhere.
Thus it seems ironic that, while so much effort goes into extraterrestrial communication, so little goes into effectively communicating with human beings here on the earth.
In that respect, SETI scientists are no different from other scientists. Most of them do not place a high premium on clarity and simplicity in writing.
No doubt this is partly because clear writing is not an integral element of a scientist's traditional training. Rather, just as one is socialized to certain work habits and theoretical perspectives by one's teachers, so does the budding scientist adopt the communicating styles of other scientists.
Another reason clarity is underplayed in scientific prose is the "commonsense" notion that scientists talk in a very difficult and precise language that precludes simplicity. That certainly may explain the use of complex terms. But such an excuse does not cover the complicated sentences and tortured strings of nouns and adjectives spilling over many a sentence in a scientific paper.
Perhaps the most intriguing attempt to understand why so many scientists lard their prose comes from a researcher at the University of Pennsylvania's Wharton School. J. Scott Armstrong has completed studies that indicate that scientists are rewarded by the system for writing unintelligibly.1 (1 J. Scott Armstrong, "Unintelligible Research and Academic Prestige: Further Adventures of Dr. Fox," Marketing Department Working Paper Series, The Wharton School, University of Pennsylvania, Philadelphia, PA, 1979.)
Since that's a very interesting sociological notion, let's take a look at Armstrong's studies and his findings in a bit more detail.
Armstrong wanted to test two conflicting hypotheses:
The first one — called the "communication-for-knowledge" hypothesis — was that if the goal of successful communication is to share information with others and if science places a premium on successful communication, then, all other things being equal, journals should prefer articles that are clearly written to those that are not. Academic conferences should look for researchers, noted Armstrong, "who have interesting studies and who could present them clearly."
The contrasting hypothesis suggested the opposite: that journals and conferences would prefer less intelligible articles to clearly written ones.
Why? Armstrong based this second hypothesis on findings from a study in which a bogus educator named Dr. Fox delivered a meaningless lecture on three occasions to 55 people, among them psychologists, psychiatrists, educators and administrators. Although the talk — entitled "Mathematical Game Theory as Applied to Physical Education" — contained nothing that made any sense, the audience members later judged the lecture to be clear and stimulating.2 (2 D.H. Naftulin, J.E. Ware Jr. and F.A. Donnelly, "The Doctor Fox Lecture: A Paradigm of Educational Seduction," Journal of Medical Education, 48, 630-635, 1973.)
Armstrong reasoned that the audience members had been confronted with a conflict: someone they regarded as a legitimate source was speaking nonsense. They resolved the conflict, said Armstrong, by rationalizing that the talk indeed had been stimulating ... although over their heads!
If such a justification for time spent was actually taking place, Armstrong reasoned, then the greater the unintelligibility, "the greater the need to rationalize about the time spent." The paradoxical result would be that the more unintelligible the paper, the more "scientific" it would be judged by its readers.
If this "Dr. Fox" hypothesis were valid, then researchers who wanted to impress their colleagues should write less intelligible papers, and journals seeking prestige should seek out as much convoluted prose as possible.
Armstrong tested his competing hypothesis in two settings:
In the first study, Armstrong looked at the relationship between the readability levels of various academic journals and their perceived prestige in their fields. Journals in the fields of management, economics and sociology were examined. Prestige rankings were obtained from scientists in the respective fields, and readability was measured by such formulas as the Flesch Reading Ease Test, a measure that uses sentence length and word length to compute a readability score.
If the communication-for-knowledge hypothesis were valid, said Armstrong, then journals that are written more clearly should be judged more prestigious than journals that are difficult to read. The Dr. Fox hypothesis would predict the opposite: the more unintelligible the writing, the more prestigious the journal's rating.
What did Armstrong find? You guessed it. Dr. Fox prevailed strongly in the management journals but to a lesser extent in the sociology and economics journals.
In his second study, Armstrong selected passages from four management journal articles and then rewrote them into varying degrees of reading difficulty without changing their meanings. Management faculty members at three universities were then given various combinations of the passages and asked to rate the "competency of the research" from which each passage came.
Armstrong's analysis of the 32 responses concluded that the sample did indeed rate the easy versions of the passages substantially lower than the harder versions. In other words, respondents felt the clearer passages came from less competent research than did the difficult passages.
Armstrong concluded from the studies that "overall, the evidence is consistent with a common suspicion: Clear communication of one's research is not appreciated."
The fact that Armstrong's two studies represent nearly the entire literature that examines relationships between clarity of communication and rewards in science is indicative of the scant attention given to this area. But, as always, things are changing. And the 1980s will see increased attention given to clarity in science communication for a number of reasons.
First, research does exist that begins to examine the effects of simpler writing on both reader enjoyment and information gain. In one study, two mass communication researchers prepared different versions of articles in three subject areas: enzymology, polymer chemistry and plasma physics. Then they gave the articles to three types of readers: low science aptitude students at a junior college, medium science aptitude students, and scientists. The most interesting finding was that, while the two college student audiences enjoyed the easier versions of the articles but not the hard versions, the scientists actually enjoyed all versions about equally. And what's more, the scientists enjoyed the easiest version more than either of the two student groups did!3 (3 G. Ray Funkhouser and Nathan Maccoby, "Communicating Specialized Science Information to a Lay Audience," The Journal of Communication, 21, 58-71, March 1971.)
But as writing is simplified, doesn't one lose information? Does simpler writing increase enjoyment at the expense of information gained?
Not according to one recent study. Another mass communication researcher asked college students to read one of three versions of a psychology journal article. The three articles varied only in language simplicity. Like the previous authors, this researcher found that reader enjoyment increased as the writing became simpler. But he also found that information gain remained the same for all three versions; readers were learning just as much from the simpler version as they were from the most difficult one.4 (4 Alan Hunsaker, "Enjoyment and Information Gain in Science Articles," Journalism Quarterly, 56, 617-619, Autumn 1979.)
Although these studies were conducted only in university settings, the findings are suggestive. It seems that even scientists enjoy simpler writing, and simpler prose — carefully done — need not result in a sacrifice of information.
The federal government in recent years also has taken a renewed interest in successful communicating through its emphasis on dissemination of research results. The National Science Foundation has served notice, for example, that it will tend to look more favorably upon research proposals that build dissemination plans into the study design. Translation: research findings need to get out not only to other scientists but also to policy makers and the interested public. The more effort you are willing to put into "popularization," the happier we will be.
Suddenly, successful communication is becoming part of the reward system.
The National Science Foundation is emphasizing clearer communication of science in other ways, too. For the first time this year, the NSF-funded Chautauqua Short Courses program for science teachers will include a course devoted to strategies for effective communication of scientific information to various publics.5 (5 Information about this course, "Science, the Media and the Public," and about the other courses being offered in the 1980-81 Chautauqua Short Courses program can be obtained from Joseph M. Dasbach, Office of Science Education, American Association for the Advancement of Science, 1776 Massachusetts Ave., NW, Washington, DC 20036.)
Finally, more scientists are developing personal interests in learning to communicate effectively. Universities have realized the need, and — as is the case with the Massachusetts Institute of Technology — some have set up writing programs for their science students. Also in some cases, the need to communicate has resulted in specialty publications, such as COSMIC SEARCH, which emphasize clarity and simplicity.
If you are interested in improving your skills in communicating with earthbound life, what can you do?
• Numerous textbooks now offer excellent instruction in clear writing. One such text, by Monroe, Meredith and Fisher, argues that scientists "ought to be good writers," that scientific analytical and organizational skills can be turned to writing if writing can be broken down into logical steps and itself treated as a science.6 (6 J. Monroe, C. Meredith and K. Fisher, The Science of Scientific Writing (Dubuque, Iowa: Kendall/Hunt Publishing Company, 1977).)
• Universities throughout the country offer courses in both science writing and technical writing (the latter can be viewed as learning to write clearly for one's peers, the former learning to write clearly for educated nonscientists). In fact, a national directory of such courses and degree programs now exists.7 (7 Sharon M. Friedman, Rae Goodall and Lawrence Verbit, Director of Science and Communication Courses and Programs (Binghamton, NY: Department of Chemistry, State University of New York at Binghamton, 1978). Copies of the directory are $4.95 prepaid from Science Communication Directory, Department of Chemistry, State University of New York, Binghamton, NY 13901.) If you are not interested in an entire course, at least you can be put in touch with individuals who specialize in science communication.
• Research in science communication is beginning to snowball. At least two publications try to keep up with such research. SCIPHERS, a quarterly newsletter for teachers of science communication, provides an annotated bibliography devoted solely to science communication studies. And Science, Technology, & Human Values, a journal devoted to the study of science in society, also picks up research reports for its annotated bibliography.
Communicators strive to share perceptions with others. The more accurately those perceptions are shared, the better. It is imperative that SETI researchers spend at least as much time groping toward successful communication with intelligent life on earth as they do trying to connect with intelligent life beyond.
SCIPHERS can be obtained by sending $8 for a one-year subscription (four issues) to Joye Patterson, Co-editor, SCIPHERS, School of Journalism, University of Missouri, Columbia, Missouri 65201.
Science, Technology, & Human Values is a quarterly review published for the MIT Program in Science, Technology and Society and the John F. Kennedy School of Government, Harvard University. Subscriptions: $10 for students and retired individuals, $12 for others and $22 for institutions. Editorial offices: Aiken Computation Laboratory 234, Harvard University, Cambridge, Mass. 02138.
Sharon Dunwoody was born in Hamilton, Ohio, in 1947. She received her A.B. degree in Journalism from Indiana University in 1969, her M.A. degree in Mass Communication from Temple University, Philadelphia, in 1975 and her Ph.D. degree in Mass Communication from Indiana University in 1978. Dr. Dunwoody has been a member of the faculty of the Ohio State University School of Journalism since 1978.
Marshall H. Cohen of the California Institute of Technology and a group of other radio astronomers at the Jet Propulsion Laboratory and the University of California, Berkeley, have proposed a transcontinental radio telescope to consist of an array of 10 dishes each 25 meters in diameter to be situated throughout the U.S. including Alaska and Hawaii. Called a Very Long Baseline Array (VLBA), this scheme would, according to the report, in effect, "transform the entire country into an immense radio telescope" and would make possible finer detailed radio maps of celestial objects including distant quasars.
Radio Amateurs interested in starting a round-table among COSMIC SEARCH subscribers should contact Frank Taylor, WB6SAE, 4312 Opal, Cypress, CA, 90630.
|
|
Technical Development and Colonization as Factors in the Long-Term Variation in Limits to Growth
By: Brian A. Tinsley
It is important to distinguish between the short term limitations to growth and the long term limitations to growth. Limitations to growth involve both limitations to growth of population, and
limitations to growth of the economy — meaning per capita consumption, standard of living, or quality of life. The short term limitations on both population and standard of living are the ones we hear most about, and are the ones we should be most concerned about. But the long term ones are worth examining also.
Short Term Limitations
It was pointed out by Malthus and by many others since then that population can increase very rapidly if there are no involuntary or voluntary limitations to it. The typical rate of increase of a healthy population not using birth control techniques and with an abundant supply of food is about 60 fold per century, or 4 percent per year.* (*Three generations per century of 8 children per couple is, on the average, 4 x 4 x 4 = 64.) This rapid rate of growth occurred in the USA in the early 19th century; and even greater rates are possible (my grandfather was one of 17 children). But it cannot be sustained for a long period because in 2 centuries it results in a 3000 fold increase. Without voluntary limitations the population will soon press against the resources, particularly the food supply, and there will be hunger, pestilence, and wars. Then the limitation of a higher death rate will stop the increase.
The same applies to the growth of the economy. For several decades in the 20th century the U.S. economy was growing faster than this rate of 4 percent per year when measured in terms of production and consumption of energy and material goods. We now see that economic growth at this rate cannot continue. The limitations involve using up non-renewable resources, such as oil and natural gas, non-ferrous industrial metals, precious metals, and minerals such as phosphorous compounds used as fertilizers. These non-renewable resources would actually decrease in availability even if the population and economy were stationary, but the increase of both advances the time when they will all be gone.
Other resources such as hydroelectric power, quantity of land available for cultivation, quantity of water available for industrial and domestic use, quantity of forest land for wood products and recreation, and ability of the ecosystem to deal with pollution, are constant, and cannot be expanded for an increasing population or increasing per capita consumption.
So we see that there are short term limitations to unrestrained growth of both population and per capita consumption. In fact, the exhaustion of non-renewable resources will probably force a decline of one or both before very long.
This is the short term picture, and I have been implying in my description of short term limitations that there is no fundamental change occurring in the way of life; no fundamental change in the technology that produces the food and material goods that determine the standard of living, and no colonization or emigration to essentially virgin lands or new habitats that the new technology makes possible.
Long Term Changes in the Limits
But in the long term we do have fundamental changes in technology and we do have colonization of virgin habitats. Whereas short term growth (over periods of about a century) will lead to a steady state, that steady state may change slowly (over thousands of years), as long term growth takes place. We should call the short term steady state the quasi-steady state.
We have had civilizations and people living in cities for 8,000 years or more, so it is relevant to wonder what the world might be like 8,000 years in the future. Will there be civilizations, and if so what sort of technology will they be based on?
The graph [below] shows the increase in world population over the last 1 million years, according to data and estimates compiled by Deevey* (*Edward S. Deevey, Jr., "The Human Population," Scientific American, Sept. 1960.) and updated from recent U.N. data. The percentage annual growth rate corresponding to a given slope varies with time and is illustrated at the top of the figure. The graph illustrates the effect of surges in the population growth rate followed by periods of low growth, and the extrapolation beyond AD 1980 is similarly designed. These effects will now be discussed.
The graph shows a surge in population starting about 1 million years ago, associated with the development of tools, clothing, and fire, which allowed an advanced hunter-gather economy to develop. Our apparent ancestors, Homo Habilis, numbering perhaps 100,000 who had been living in tropical Africa., were able to support a greater population locally with this development, but more important, were able to move out into other habitats, including the fringes of the ice sheets in northern Europe and Asia, killing large mammals and using their skins for clothing.
This led eventually to populations at home in the ice of Siberia, and then Alaska, which were then able to expand south through North and South America.
About ten thousand years ago the invention of agriculture and irrigation in places such as the Middle East, China and Middle America permitted a population surge to a new level. The technological capability to cut down forests led to the development of central European population centers.
A few hundred years ago in the eighteenth and nineteenth centuries, the advent of the industrial revolution, with improved transportation, mechanization of agriculture, improved hygiene and medicine gave rise to a surge which has not yet been limited.
It is the development of technology in each case which has raised the limits of the population which the resources of the world can support. In general the technological development has raised the per capita income and consumption as well as the total population, especially where voluntary family limitation results in a population growth rate less than that of the economy.
The short term population and consumption pressure will often exhaust non-renewable resources, or resources which could be husbanded but are not. Such a situation occurred in England in the 18th century, when the consumption of wood for metallurgy resulted in the forests being cut down, resulting in a great scarcity of wood. This forced people to turn to coal (in spite of much opposition) which led to much more efficient industry that hearlded [sic; "hearlded" should be "heralded"] the industrial revolution and a surge of population growth.
Resource Substitution
The replacement of wood by coal was an example of resource substitution. Growing crops and breeding animals instead of hunting and gathering a wild population was another. But the cultures responsible for exhausting a resource do not necessarily have the social or technical capabilities for making such a substitution. The failure of the Roman civilization was partly due to the overcropping and exhaustion of the North African food growing areas without substitution.
The present short term crisis due to the depletion of non-renewable energy sources and mineral sources is likely to lead to declines of standards of living, and even population declines, unless there is resource substitution. Nuclear fission power and coal can provide stop-gap help for a century, but the (1,000 year) long term energy source will have to come from controlled fusion and/or solar power (photovoltaic, solar collector, or biomass conversion).
There will be large increases in the prices of heavy metals, which will probably result in very much greater recycling and much less loss. But plastic, ceramic and glass structures will have to largely replace metal. One would expect to see goods of much greater durability replacing the current variety designed for a short lifetime and rapid obsolescence.
The shortage of fertilizer (phosphorous and energetically expensive nitrogenous based fertilizer) will probably result in a shift from consumption of meat to consumption of cereals (which is a more efficient food cycle) unless biological engineering can produce new varieties of plants and animals that yield a much higher food return per unit of energy investment, or of phosphorous consumption, than at present.
The use of energy for home and office heating and cooling and for transportation will have to be reduced. This means redesigned houses with better thermal insulation — possibly in the form of compact apartments close to places of work (also better insulated) and the use of solar heating and cooling. Automobiles will have to run on fuels such as alcohol or hydrogen, produced by the biomass conversion, solar power or fusion, or from batteries, charged at night from central power plants, and they will be much smaller and energy efficient. Unless these rather fundamental technological changes are made, the population levels will decline, perhaps catastrophically.
With the increasing shortages and widespread pessimism, the complex structure of the economy could break down, and the food production, processing, distribution and marketing process could break down, along with water, electricity and heating oil and gas distribution. The deaths due to malnutrition and disease could amount to a significant fraction of the population. An internal or foreign war would also be likely. But it is unlikely, even with a war, that the population would go to zero. Even with 90 percent deaths in a nuclear war, population levels would be back only to levels 1,000 years ago, and with the recorded scientific knowledge and plenty of examples of its application, technology and population might soon press upwards again.
The situation at present represents a competition between the restrictive tendencies of depletion of non-renewable resources, and the growth tendencies permitted by continual technological development. At present the growth tendency due to development is prevailing, with an annual world growth rate of 2 percent, the highest in history. In the face of severe resource depletion in the next 50 years, the situation seems inevitably about to reverse. At least some cultures would seem to have the social and technological capabilities to take decisive action (similar to a mobilation for war) to cope with the need to substitute resources and further limit birthrates, but much of the success of such action will depend on the seriousness with which the situation is regarded, and the willingness of people to change their lifestyles without a large amount of political friction and wasteful fighting to get the last of the resources which are bound to run out anyway.
Colonization
Now I want to discuss the effect of colonization of new habitats. The departure from Africa of members of Homo Habilis for northern Europe and Asia a million years ago probably did not relieve short term population pressure in Africa much. But the overall effect was the development of a new center of population, very likely several times larger than the African center, and most importantly, with a higher level of technology.
Similarly the colonization of North America by Europeans, while it did not relieve population pressure in Europe much, again resulted in the development of a new center of population, comparable to that of Europe, and most importantly, it stimulated the development of new technology for new conditions.
The next step would be in the future, with the development of small self-supporting colonies in space. This seems highly speculative now, but much technological progress can be expected on a 1,000 year time scale, which is short compared to the scope of this essay. In space, solar energy would be readily available, and sufficient sources of raw materials would probably be found in asteroids and planetary satellites. The development of this type of economy would be significant, since if it was successful in being self-sustaining, then it could eventually result in units leaving the solar system under thermonuclear power, and slowly moving out to colonize the galaxy, over a period of 1 million years or so. There are about 100 billion stars in the galaxy, and there are probably planetary systems near a large fraction of them that are a source of raw materials, with the star available for energy, so in this sense the long term limits to growth would be pushed back far beyond the present ones.
The percentage annual growth rate of the total human population even with space colonization is never likely to be as large as the current rate of about 2 percent (unless almost all of the human population is wiped out and the growth starts from a low base level again). The reason is that according to the laws of physics it would be impossible for a wave of colonizing spacecraft to move out through the galaxy faster than the speed of light.* (*Assuming colonies produced a uniform population density in the galaxy, the fractional increase per unit time of volume of space populated by a wave of colonizers moving at the speed of light (c) is equal to 4πr2c/(4/3)πr3 = 3c/r where r is the radius of the volume colonized. Thus, the fractional rate would be 2 percent per year when r equals 150 light-years, but less than 2 percent if r is greater. Furthermore, actual velocities would be well below the speed of light reducing the rate even more. Thus, it seems that the current human population increase rate is unlikely to be ever again attained. At 1 percent of the speed of light, a few million years would suffice to complete the colonization.)
Of course, it is quite possible that humans would not be the only intelligent species colonizing the galaxy in this way. In that case growth of a different sort — intellectual growth above that developed by just being in space — would very likely result from the meeting of the two cultures — even if the population and economic growth were thereby limited.
Thus, the effort to establish habitations in space should be encouraged. Even though the cost of putting man in space is significant, so was the cost to the European courts of the 15th and 16th centuries of sending Columbus (and others) out to explore the western Atlantic. We cannot expect to predict the most important benefits that would accrue from a prolonged effort in establishing man in space. Some less important ones would be a great advance in understanding the nature of the universe, of which the phenomena on earth are only an insignificant part; the tapping of new energy sources, possibly including the production and controlled feeding of miniature black holes; and a new realization of the vast range of capabilities of human beings to live satisfying lives in unconventional environments.
While we would not expect the colonization of space to have an immediate effect on the pressure of population against resources back on earth, in the long term it probably would be beneficial as new technology developed in space was applied back on earth.
This sort of transfer between colonies and parent societies has been a pattern during the last million years. Surely we owe to future generations this opportunity for future growth and development of the human species.
Relevance to Present Problems
Thus we have to realize that Malthus was right in the sense that there will always be pressure of population on resources unless there is a voluntary limitation on the population growth rate (which can be for quite private reasons, such as the preference for a life free of the burden of caring for children, or the desire to give only one or two children a better start in life than is possible for many). A generalization of Malthus' Law would read "The unchecked rate of natural increase of population is so high that it can only temporarily be matched by the rate of increase of the economy. In general, unless population growth is limited voluntarily, below or at the rate of increase of the economy, it will be limited by involuntary mechanisms, with a decrease in the quality of life."
In conclusion, the advancement of science has allowed us a much longer time perspective than that available to Malthus, and we see that with technological development it is possible to substitute new resources for old, and that there is no particular reason why development with a slow population growth cannot continue, provided intelligent planning for the future is made, and provided the investment in scientific research and technological development is continued, and further that societies are willing to plan for and accept the disruption that will inevitably accompany the change.

Brian A. Tinsley was born in New Zealand in 1937. He received his B.S. degree in 1960 and Ph.D. degree in 1963 both from the University of Canterbury, New Zealand. Dr. Tinsley has worked at the University of Texas at Dallas (originally the Southwest Center for Advanced Studies) since 1963. He teaches Physics and Astronomy and is involved in research on upper atmospheres, especially interactions between their high altitude neutral atomic coronas and trapped ions.
He currently operates photometric equipment in the Peruvian Andes; Mt. Haleakala, Hawaii; and at MacDonald Observatory, Texas, to measure optical emissions from energetic neutral atom precipitation. He is planning to make similar measurements from a spectometer on Spacelab in 1984.
|
In Search of Planets
(The Extrasolar Planetary Foundation Report)
By: George D. Gatewood
Perhaps even more basic than the question of extraterrestrial life is that of extrasolar planets. It is hard to imagine life evolving without a planetary home, and so far our Solar System seems to have provided only one such abode. Thus, extraterrestrial life would seem to require extrasolar planetary systems. If neighboring planetary systems exist, and if they contain inhabitable planets devoid of life evolved from their own ecosystems, the question of extraterrestrial life will need redefinition: by this I mean that the extraterrestrials may come to be understood to be none other than humans from Earth who have colonized other planets. Although the distances are vast, they are not insurmountable and our descendants will some day be able to venture to the stars.
The advent of new astronomical detectors has made the first step in this adventure possible now. Small groups of scientists working at several institutions across the United States are developing the first instrumentation capable of discerning which stars harbor their own planetary systems. To help these groups test new ideas and concepts, and to help them to begin the observational programs that will point the way for the future, we have formed the Extrasolar Planetary Foundation. With a Board of Directors composed of astronomers and lay persons with related backgrounds, we hope to act as a focal point for those who would like to give this new effort a measure of support. Our experience suggests that the number of people who would like to participate in this effort is large enough to raise an endowment of several hundred thousand dollars. The interest earned by such a fund would become a perpetual source of support, providing new instrumentation and providing the long-
term stability necessary for the observational programs that will be required.
The editors of COSMIC SEARCH support our effort and have offered us this space to begin a regular column. We will here report to you further on both our foundation and the research we hope to support. For further information, write Extrasolar Planetary Foundation, Observatory Station, Pittsburgh, Pennsylvania 15214. Your tax deductable patronage will be greatly appreciated.
George D. Gatewood
Chairman of the Board
Extrasolar Planetary Foundation
Dr. George D. Gatewood is Director of the Allegheny Observatory of the University of Pittsburgh.
|
People
By: John Kraus
Carl Sagan, of Cornell University, has produced a new 13-week television series "Cosmos" being presented this fall over Public Television. The production, funded by $8 million in grants, is a personal voyage through the cosmos touching on planets, stars, evolution, SETI and a host of other topics in an entertaining, instructive and responsible format. "Cosmos" is a welcome addition to the all-too-few outstanding science-education presentations available to the viewing public and should help to awaken a cosmic awareness in many people.
"Cosmos" is television at its very, very best.
Dr. Sagan is a member of the Editorial Board of COSMIC SEARCH.
The Reverend Theodore M. Hesburgh, President, University of Notre Dame, was presented the Sylvanus Thayer award of the U.S. Military Academy on September 11, 1980, in New York City. The award, representing the Academy's motto, "Duty, Honor, Country", was established in 1958. Dr. Hesburgh is a member of the Editorial Board of COSMIC SEARCH.
Richard E. Berendzen was invested as the 11th president of the American University, Washington, D.C., on September 18, 1980. Dr. Berendzen is a member of the Editorial Board of COSMIC SEARCH.
Norman Cousins of SATURDAY REVIEW has been appointed Adjunct Professor of Psychiatry and Behavioral Sciences at the University of California, Los Angeles. Dr. Cousins recently authored a new book "Anatomy of an Illness" (W.W. Norton) which is enjoying a wide sale. Dr. Cousins is a member of the Editorial Board of COSMIC SEARCH.
George E. Brown, Jr., Congressman from California, has introduced a bill in the 96th Congress (H.R. Bill Number 6304) designed:
"To establish the national space policy of the United States, to declare goals of the Nation's space program and to provide for the planning and implementation of such program."
Further, under Section 5, titled "Program" the bill reads:
"The goals in space science to be achieved ... are the continuation of research in the life sciences to insure human health, safety, and effective performance in space flight, to further use the space environment and space technology in the advancement of knowledge in medicine and biology and to understand the evolution and distribution of life in the universe. (Italics ours).
Carl Sagan of Cornell University and Bruce Murray of the Jet Propulsion Laboratory have announced the formation of The Planetary Society, a nonprofit organization aimed at bringing together professional and amateur space enthusiasts as a visible and influential group. Persons interested in joining should write to: The Planetary Society, Box 3599, Pasadena, CA, 91103.
George Gatewood of the University of Pittsburgh and Frank Drake of Cornell University have announced the formation of the Extrasolar Planetary Foundation aimed at mustering research support for the detection of planets which may be circling stars beyond the solar system. Dr. Gatewood will write a regular column for COSMIC SEARCH on the activities of the Foundation. His first column appears in this issue on page 12.
Robert A. Frosch has announced that he was resigning as Administrator of the National Aeronautics and Space Administration (NASA), effective January 20, 1981, to become the first president of the American Association of Engineering Societies, a federation created early this year comprising the major engineering societies of the United States. Dr. Frosch became the fifth head of NASA in June 1977.
A four-day seminar on "Macroengineering" was held at the Massachusetts Institute of Technology last summer. The slackening pace of the U.S. space efforts came under discussion. Some conferees voiced the opinion that large-scale projects will be difficult to achieve because of a growing political and corporate unwillingness to attack mankind's fundamental long-term problems.
A week-long conference in Rome featured astronomy and astronautics. Held last summer as a part of the Second International Festival of Poets it explored the philosophical consequences of mankind's surge into space. Whereas interest in space appears to be waning in the U.S. it is waxing in Europe. For example, the U.S.S.R.'s space program continues to move steadily ahead with ambitious plans for the 1980's.
John N. Wilford delved deeply into the divergent paths of the U.S. and Soviet space programs in "Science Times" (New York Times) for October 14, 1980. He reported that whereas the Soviet efforts aim toward establishing a permanent space station using, at least so far, older techniques, U.S. efforts center around the problem-ridden high-technology space shuttle.
Trudy E. Bell published a comprehensive report on "American Space Interest Groups" in the September 1980 issue of "Star and Sky". A table covering 4 pages gives detailed information and statistics on over three dozen American space organizations both professional and citizen supported.
Spectrum, the monthly publication of the Institute of Electrical and Electronics Engineers, carried 11 articles in the September 1980 issue on the general topic "They Said It Couldn't Be Done (and It Hasn't)". These articles give in-depth discussions of 11 important technological problems where breakthroughs and success have not been achieved. Some of the problems considered are the superconducting power cable, the electric automobile, magnetohydrodynamic power generation,
collision-proof airspace, an electronic "human eye", global video conferences, and a no-downtime computer. Who said that there are no challenges facing new electrical engineering graduates?
An UHURU Memorial Symposium is being held on December 13, 1980, at NASA's Goddard Space Flight Center to discuss the past, present, and future of x-ray astronomy. The symposium marks the 10th anniversary of the launching of UHURU (pronounced oo-who-ru), the first orbiting x-ray observatory.
The Astronomical Society of the Pacific (A.S.P.) is sponsoring a weekly syndicated astronomy column in an attempt to increase the number of clear, reliable astronomy reports available to newspaper readers. Called "Exploring the Universe" the weekly series concentrates on the most current and exciting concepts and discoveries in astronomy. If you are interested in more details about this column and how to get your local paper to carry it, write to Andrew Fraknoi, A.S.P., 1290 24th Ave., San Francisco, CA, 94122.
JK
|
The SEnTInel
By: Robert S. Dixon
What Caused the Dinosaur's Extinction?
Many reasons have been suggested in the past to explain why the dinosaurs suddenly became extinct
about 65 million years ago. Among these are a sudden climate change, a reversal of Earth's magnetic field, and a nearby supernova. Actually the dinosaur extinction is only the most recent in a series of great extinctions that have occurred five times since the time of the oldest fossils, or about once every 100 million years. During these extinctions entire groups of plants and animals disappeared completely, yet others survived. For example, during the dinosaur extinction most of the water-living reptiles, all of the flying and large land reptiles, and many kinds of sea plants died out. But mammals, amphibious reptiles (like crocodiles), snakes and land plants survived. Any theory which successfully explains these events should explain not just one extinction but all of them, and should explain why some life forms died out while others lived on.
Recently acquired geological evidence now points strongly toward the hypothesis that the extinctions were caused by asteroids colliding with the earth. Luis Alverez, Walter Alverez, Frank Asaro and Helen Michel of the University of California at Berkeley explained their findings in the June 6, 1980 issue of Science.
Astronomers calculate that an asteroid of 10 km diameter or larger will collide with the earth about once every 100 million years, in good agreement with the frequency of the great extinctions. A 10 km diameter asteroid collision would make a crater about 200 km across. Three known craters exist on the Earth today that could have been caused by such impacts. The dust clouds thrown up by such an impact would quickly enter the stratosphere and be distributed by the wind to completely encircle the earth. This would effectively block out most of the sunlight and cause photosynthesis in plants to stop. As the plants died out, so would the animals that eat the plants, including most of the dinosaurs. As these animals died out, so would those who prey on them (the meat-eating dinosaurs).
The only survivors would be those who could temporarily exist on other food sources. The only mammals of that era were small, rodent-like creatures that could eat roots, nuts, seeds and other non-perishable food. Thus, they survived. Also in turn those animals that preyed on the small mammals (snakes and crocodiles) could survive. After several years the dust clouds would settle back to earth and the sun would reappear. Then the land plants which had lapsed into dormancy could spring up again from seeds or by sending forth new shoots from their roots.
It is useful to extrapolate the effect of an asteroid collision from the effects of the largest explosion in modern history; that of Krakatoa. Krakatoa is an island volcano in Indonesia that exploded with extreme violence in 1883. Although far smaller in its effects than an asteroid collision, Krakatoa did in fact throw up clouds of dust that encircled the earth. Brilliant sunsets caused by the dust occurred worldwide soon after the explosion. The sunsets lasted several years, and then stopped, indicating that the dust had settled back down to the earth. Thus, it may be reasonable to believe that an asteroid collision would cause effects similar to those required to cause the great extinctions.
Reasonable as this scenario may seem, it does not prove that an asteroid collision did in fact occur 65 million years ago, just at the time of the last great extinction. It is to this point that the Alverez group directed most of their effort. They studied the rock layers that were deposited at that time, in an effort to learn if they differed in any significant way from their neighboring older and newer layers. To eliminate local variations, they studied those layers at widely separated outcroppings in Italy, Denmark and New Zealand.
As the Earth originally formed and cooled from its molten beginning, certain groups of chemical elements migrated toward the center, leaving the outer crust relatively depleted in these elements, compared to that of the solar system as a whole. For example, the rare metal iridium is much less common in the Earth's outer crust than it is in asteroids, meteorites and interplanetary dust. The Alverez group reasoned that if an asteroid struck the earth, the dust it threw up would contain more iridium, relatively speaking, than is found in the average soil on Earth. As the dust settled, it would enrich the rock layers currently being deposited at the bottom of the ocean, so that there would later be a narrow layer of rock that contained an anomalously high concentration of iridium.
When the Alverez group analyzed the rock layer that was created at the time of the dinosaur's extinction, they found that the concentration of iridium was 50 times greater than in any neighboring rock layers. This is strong evidence that the earth had a sudden inflow of extraterrestrial material, that lasted only a short time, and occurred at the same time the dinosaurs became extinct. An asteroid collision seems to best explain all the observed facts.
The wider implications of all this on the evolution of life in the universe are unclear. It seems likely that the extinction of the dinosaurs left the surviving mammals with fewer competitors and predators, thereby allowing them to more rapidly expand their habitats and evolve to occupy more ecological niches. On the other hand, this might have occurred eventually anyhow. If occasional great extinctions caused by asteroid collisions are a necessary condition for intelligent life to evolve, then the frequency of intelligent life is dependent on the frequency of available asteroids. We do not know if our solar system is unusual in having an asteroid belt, so we can only speculate as to whether the work of the Alverez group increases or decreases our estimates of the frequency of intelligent life in the universe.
Faster-Than-Light Motions Observed in Radio Sources
In recent years it has become possible to record radio signals of a celestial object simultaneously at a number of different radio telescopes located in various places on the earth, and then later combine the data from all the telescopes to simulate what would have been observed with one huge radio telescope that is nearly as large as the Earth. This observing technique is known as a very long baseline interferometry, or VLBI. It can provide angular resolution of 1/1000 of a second of arc, which is significantly better than can be achieved with conventional optical telescopes.
This extreme resolving power has been used to study objects whose angular diameter is very small, indicating that the objects are either very small in size, very far away, or both. The most exciting discovery made thus far using this technique came about from a program that monitored the finely-detailed structure of about 10 such objects over a period of several years. This effort was summarized by Alan Marscher of the University of California at San Diego and John Scott of the University of Arizona, in the April, 1980 issue of the Publications of the Astronomical Society of the Pacific. Most radio sources never change their observable shape, but amazingly enough, half of the compact objects studied do change, and in a most dramatic way. Several components of these objects appear to be moving at speeds greater than that of light, with respect to one another (such motions are called superluminal). Their speeds are not just marginally faster than light, but range up to twenty times that of light!
Clearly something is wrong here, since Einstein's Theory of Relativity says that nothing can move faster than the speed of light. But we have to be careful here and point out that "nothing" means "no thing that has mass." It is quite possible for some kind of an effect to move faster than the speed of light. In fact it is fairly easy to create an effect that moves faster than light. Suppose you shine a flashlight on the wall and rapidly change the direction in which you point it. The spot of light on the wall will move very quickly. By a mere flick of the wrist you can move the spot 100 kilometers per hour or more, right in your own room. The important thing to note here is that the spot itself has no mass; it is not a material "thing"; it is only an observable "effect". It's fairly easy to see that to achieve higher spot speed, all we have to do is put the wall further away or move the flashlight faster. To reach the speed of light, we only have to fasten the flashlight to the shaft of a common electric motor (this will spin the spot in a circle 3,600 times a minute) and shine it on a mountain range or cloud bank 800 kilometers away. Of course, this simple experiment has some practical difficulties, but it serves to illustrate the point.
Using this concept of "effect" motion, astrophysicists have developed several different explanations of why the radio source components seem to be moving so fast. So far, no one is really sure which explanation is correct, and only by continual monitoring or these objects over a period of several more years will astrophysicists be able to decide on the correct explanation.
The Universe as a Black Hole* (*Contributed by David Raub)
In the previous issue of COSMIC SEARCH (Summer, 1980), two different kinds of black holes were discussed in an article appearing in the SEnTInel column. One of these, "classical" black holes, is formed by the gravitational collapse of dying stars at least three times as massive as our sun. These black holes can be very large and are the kind usually referred to in today's popular science literature.
The other type of black hole, "primordial" black holes, is postulated to have formed during the
early moments of the Big Bang.
One interesting attribute of both classical and primordial black holes is that they are not completely "black". Rather, they both emit thermal radiation at a rate inversely proportional to their size (the smaller the black hole, the higher the temperature). This thermal radiation is caused by quantum mechanical effects which result in spontaneous "particle creation" near the observational boundary of the black hole. These newly created particles occasionally tunnel out through this boundary and might be seen by observers like us as ordinary thermal radiation.
Recently Stephen Hawking of Cambridge University and an associate, G.W. Gibbons of the Max Planck Institute for Theoretical Physics and Astrophysics, have extended the ideas of black hole thermal radiation and quantum particle creation to the universe as a whole. In the black holes discussed above the strong gravitational field produced by a collapsing mass is so strong that light emitted from the mass is dragged back and cannot be seen by observers outside the black hole. The boundary
of this region of unobservability is referred to as the black hole "event horizon".
Event horizons of a different kind occur in certain cosmological models where the rate of expansion of the universe is so rapid that it greatly exceeds the strength of the gravitational field to cause collapse. The effect of this rapid expansion is that for each observer there are regions of the total universe from which light can never reach them. Hawking and Gibbons, writing in the May 15, 1977 issue of Physical Review D (Volume 15, Number 10), call this region the "cosmological event horizon of the observer". In a sense, Hawking and Gibbons are really discussing the event horizon associated with a third kind of black hole — the universe itself.
Such cosmological event horizons apparently have many formal similarities to ordinary black hole event horizons. One might therefore expect that with the existence of a cosmic black hole, particle creation with a corresponding thermal radiation would also occur near its event horizon. The authors' calculations show that this is indeed the case. Observers such as us would detect thermal radiation with a characteristic wavelength of the order of the Hubble radius (e.g., a wavelength
which is the width of the entire observable universe). This would correspond to a temperature of less than 10-28 degrees kelvin so that it is not of much practical significance. However, it is important conceptually because it shows that black hole thermodynamical arguments can be applied to the entire universe.
Hawking and Gibbons regard the area of our cosmological event horizon as a measure of our lack of knowledge about the remainder of the universe beyond our observational limit. If one absorbs thermal radiation coming from this event horizon with a hypothetical particle detector, one gains energy and entropy at the expense of the unobservable regions of the universe beyond. Therefore, the area of the cosmological event horizon decreases. As the area of the horizon goes down, the temperature of the thermal radiation emitted by particle creation also decreases so the cosmological event horizon returns to a stable condition. However, if we choose not to activate our particle detector and subsequently do not disturb any radiation, there is no change in the temperature nor in the area of our cosmological event horizon. This astounding fact shows that particle creation and the back reaction associated with it seem not to be defined independently of the observer. Rather, we find that quantum particle creation and its subsequent thermal radiation are observer-dependent (e.g., they depend upon whether the observer chooses to activate his particle detector or not).
Hawking and Gibbons discuss the implications of this notion of observer-dependence at the end of their fascinating paper and conclude that one must adopt a seemingly bizarre interpretation of quantum mechanics called the Everett-Wheeler interpretation to account for these amazing facts. This interpretation views the universe as constantly splitting into a stupendous number of alternative parallel worlds, each world representing a possible course our world has taken as the result of physical interactions between the universe's many microscopic components in the past. In actuality all of these parallel worlds really exist, but we can only experience one of them.
To see what this "parallel world" concept implies, one need merely note that because every cause, however small or large, may ultimately affect the evolution of the entire universe, it follows that every quantum transition taking place on every star, in every galaxy, in every remote corner of our universe is constantly splitting our local world on earth into an infinity of copies (including copies of us as well!).
This novel interpretation of quantum mechanics is gaining increasing acceptability among scientists these days, especially among physicists engaged in research associated with the quantum mechanics of the whole universe.
Since the Search for Extraterrestrial Intelligence (SETI) is concerned with the detection of something which could indicate the existence of other intelligent life beyond the earth, experimental verification of the conclusions drawn by Hawking and Gibbons (and hence proof of the validity of the Everett-Wheeler interpretation of quantum mechanics as well) would mean that the lucky scientist making such a discovery would not only prove the existence of a single extraterrestrial civilization, but rather an infinity of civilizations existing in different parallel universes (even though he may never hope to communicate with any of them).
|
ABCs of Space
THE UNIVERSE IN SEVEN STEPS
By: John Kraus
The topic for this issue is taken from a few pages of my new book OUR COSMIC UNIVERSE (Cygnus-Quasar Books).* (* Copyright © 1980 John D. Kraus.)
We live in a vast and mysterious universe. We are gradually learning something of its enormous size and of the rich variety of objects it contains. By way of introduction let us take a brief excursion across it.
The earth is a spinning sphere or planet which, with eight other planets, revolves around a star we call the sun. Beyond the sun and its planets are other stars, the nearest of which is more than 4 light-years away. That is, it is so far it takes light, traveling 300,000 kilometers per second, more
than 4 years to travel the distance. The sun and 100 billion other stars constitute our galaxy, a huge flat, disc-like aggregation of stars turning like a great wheel in space. Beyond our galaxy are 100 billion other galaxies which fill our universe.
With this brief overview in mind let us go back and take the trip in a more leisurely fashion, this time in seven steps:
Step 1. The Earth and Its Rings
Step 2. The Earth-Moon System
Step 3. The Solar System
Step 4. The Solar Neighborhood
Step 5. Our Galaxy
Step 6. The Galactic Neighborhood
Step 7. The Universe
Now let us consider each of the steps in more detail.
Step 1. The Earth and Its Rings. The rings of Saturn are its best known feature. From close-ups radioed back from Voyager 1, its three principal ring zones appear to consist of many narrower ones, perhaps hundreds of them, looking much like the grooves on a phonograph record. Not only Saturn, but Jupiter and Uranus have rings.
The earth also has rings, at least four of them: two Van Allen rings (or belts), a Clarke ring (or orbit) and extending farthest out, an auroral ring. These rings were described in ABCs of Space in the last issue of COSMIC SEARCH (Summer 1980, Serial number 7.).
In this issue we will continue the trip through Steps 2, 3 and 4 and then complete our journey across the universe via Steps 5, 6 and 7 in the next issue.
Step 2. The Earth-Moon System. Light or radio waves can travel the distance to the moon in about one second. This is the distance reached by manned exploration.
Closer to the earth (at about 36,000 kilometers) are the man-made synchronous satellites travelling around the earth once per day, staying fixed or synchronized above a point on the earth's equator as explained in Step 1.
Step 3. The Solar System. The sun, its nine major planets and 1000s of minor planets or asteroids constitute the solar system. The sun is a nuclear-powered star about one and one-half million kilometers in diameter. It is 6 billion kilometers from the sun to the outermost planet, Pluto. Light or other electromagnetic waves travel this distance in 51/2 hours. The earth-moon system is relatively close to the sun at a distance light traverses in 8 minutes.
Unmanned probes have penetrated to the orbit of Saturn or one-third of the way to Pluto. The earth-moon system of Step 2 must be reduced by a factor of 10,000 to correspond to the scale of the solar system (Step 3).
The dots showing the sun, earth and other planets are relatively too large. To represent their proper sizes they should be at least 100 times smaller in diameter.
Step 4. The Solar Neighborhood. The sun and some of the closer stars form the solar neighborhood. There are a dozen stars closer than about 10 light-years, the nearest being about 4 light-years; however, with the exception of Sirius, Alpha Centauri and Procyon, they are too faint to be seen with the unaided eye.
The solar system must be reduced by a factor of 17,000 from the Step 3 diagram to be the correct size in the solar neighborhood diagram. The dot for the solar system (diameter equal to Pluto's orbit) should be at least 1000 times smaller, while the star dots are a million times too big.
|
In Review:
By: John Kraus
"Reflections", a 16-minute sound-color film by David Hoffman.
"One hundred sixty one sunrises, one hundred sixty one sunsets in 10 days — the speed — the silence — the
incredible spectacle of the bright blue earth below on which we are all riders together — a oneness with the whole earth — a fantastic experience."
With words like these, Russell "Rusty" Schweickart takes you for a thrilling ride with him as he skims above "the womb of the earth we call the atmosphere" sharing with you his thoughts and emotions in a deeply moving, extremely impressive presentation. Rarely have the feelings of an astronaut been so eloquently expressed. The philosophical effects of spaceflight and the enormous potential of this new arena for all mankind are made apparent.
"Rusty" Schweikart will never be the same and he has an important message to share. Viewers can't help but acquire more of a cosmic perspective.
The film has taken first place at a number of film festivals. It is available in 16 and 35 millimeter widths as well as on video cassettes. Produced by David Hoffman, the film is geared to all audiences — schools, colleges, churches, private gatherings, etc. Anyone interested should contact: David Hoffman, 100 Pascal Avenue, Rockport, Maine 04856. (Tel. 207-236-8506).
John Kraus
|
Miscellaneous Items
Webpage Table of Contents (Bookmarks) (Internal links to categories of items in this webpage)
Information About the Publication (Editorial Board, Editors, Table of Contents)
Editors, and Others Involved in the Publication
Editor: John Kraus, Director, Ohio State University Radio Observatory.
Co-Editor: Robert S. Dixon, Assistant Director, Ohio State University Radio Observatory
Assistants: Alice Kraus, Jim Arthur
Editorial Board
- Richard Berendzen, President, The American University
- John Billingham, Director SETI Program, NASA-AMES Research Center
- Ronald Bracewell, Director, Radio Astronomy Observatory, Stanford University
- Thomas A. Clark, NASA-Goddard Space Flight Center
- Arthur C. Clarke, Sri Lanka, author of "2001, A Space Odyssey"
- Norman Cousins, Chairman, Editorial Board, SATURDAY REVIEW
- Frank D. Drake, Director, National Astronomy and Ionosphere Center (Arecibo), Cornell University
- Robert E. Edelson, SETI Project Manager, Jet Propulsion Laboratory, California Institute of Technology
- Donald S. Hall, Director, Strasenburgh Planetarium, Rochester, New York; Past President, International Planetarium Society
- Theodore M. Hesburgh, President, University of Notre Dame
- Nikolai Kardashev, Space Research Institute, Academy of Sciences, Moscow, USSR
- Philip Morrison, Physics Department, Massachusetts Institute of Technology
- Bernard Oliver, Vice President, Hewlett-Packard Company; Director of NASA-Ames Cyclops Project
- Cyril Ponnamperuma, Director, Laboratory of Chemical Evolution, University of Maryland
- Martin Rees, Director, Institute of Astronomy, Cambridge University, England
- Carl Sagan, Director, Laboratory for Planetary Studies, Cornell University
- Walter Sullivan, Science Editor, New York Times
- Vasevolod S. Troitsky, Radiophysical Scientific Research Institute, Gorky, USSR
- Sebastian von Hoerner, National Radio Astronomy Observatory
About COSMIC SEARCH
COSMIC SEARCH is published quarterly (Winter, Spring, Summer, Fall) by Cosmic-Quest, Inc. Copyright © 1980 by Cosmic-Quest, Inc. All rights reserved. Cosmic Quest, Inc., is a non-profit educational-scientific (tax-exempt) organization.
Subscription price: $10 for 4 issues in U.S. (and possessions), $13 elsewhere. Single copies: $2.50 in U.S. (and possessions), $3 elsewhere.
Address subscriptions and all other correspondence to: Radio Observatory, Box 293, Delaware, Ohio 43015.
Second-class postage is paid at Delaware, Ohio, and at additional mailing offices.
Opinions expressed by persons writing in COSMIC SEARCH are their own and do not necessarily reflect the opinions of the editorial staff.
Statement of Ownership, Management and Circulation
Front Cover
Distant galaxy? No. Storm on Jupiter? No. Big White Spot on the Earth? Yes! Hurricane Allen filling the Gulf of Mexico at noon on August 8, 1980. Tick marks indicate the coasts of Texas at upper left and of Yucatan at lower right. (Photo courtesy NOAA/NESS). See article by James E. Lovelock starting on page 2.
Back Cover
"Big Ear", the 110-meter radio telescope of the Ohio State University. A montage by Jim Arthur.
Table of Contents (in magazine)
Item | Pg |
The Recognition of Alien Biospheres by James E. Lovelock | 2 |
Strategies of Searching for Extra-Terrestrial Intelligence (Part Two) by Nikolai Kardashev | 4 |
SETI by Frank D. Drake
| 7 |
Why is Scientific Writing Unintelligible? by Sharon Dunwoody | 8 |
Technical Development and Colonization as Factors in the Long-Term Variation in Limits to Growth by Brian A.Tinsley | 10 |
In Search of Extrasolar Planets (EPF Report) by George Gatewood | 12 |
To All Cosmic Searchers
In its first two years, COSMIC SEARCH lost at the rate of about $2000 per month until our debt reached $50,000. We could not continue under such circumstances and we seriously considered ceasing publication and declaring bankruptcy.
However, we decided to continue for the present but with such changes as required to hold costs below income. Accordingly, many economies have been effected. Two of the more significant ones are:
(1) giving up our one and only paid employee and going back to a completely volunteer operation, and
(2) reducing the number of pages per issue.
We hope these will be temporary.
There are many ways in which you can help:
(1) by renewing your subscription promptly
(2) making gift subscriptions
(3) telling others about COSMIC SEARCH and urging them to subscribe and
(4) making a gift or donation.
COSMIC SEARCH is a non-profit scientific-educational magazine which we believe fulfills an important purpose. In
response to a questionnaire sent out recently to a random sampling of our readers, the most frequent statement in almost identical words was: "COSMIC SEARCH is a fantastic magazine. Please keep it going".
We will try but we need your help.
Owing to space limitations it is necessary to omit the "Letters" and "College Courses" departments from this issue.
However, two new columns have been added, one by Frank Drake and the other about the new Extrasolar Planetary Foundation by George Gatewood.
Because of the small all-volunteer staff and the need to wait until there was money on hand to pay the printing and associated costs, this issue is late. Hopefully, subsequent issues will be more on schedule.
John Kraus
Thank You!
COSMIC SEARCH expresses sincere thanks to the following donors who are helping to make sure that the story of SETI and mankind's future continue to be told in an interesting and factual way.
Planetary Donors
Robert J. Allen, Blythdale, Missouri (2 years)
Jane L. Brooks, Adelaide, S. Australia (2 years)
Amahl S. Drake, Ithica, New York
James C. Killman, Sherman, Texas
David M. Laida, Sierra Vista, Arizona
Arthur J. Morgan, New York, N. Y.
A. V. Shaver, Winchester, Virginia
Paul Simons, Sheboygan, Wis.
Eric W. Six, Iowa City, Iowa
John B. Theiss, Tucson, Arizona
Dennis Wildfogel, Pomona, N.J.
One anonymous
Stellar Donors
George L. Douglass, II, Reno, Nevada
David C. Halley, Colonial Hts., Virginia
Paul Simons, Sheboygan, Wis.
John Teetor, Marion, Ohio
C.L. Turnage, Camarillo, California
Cosmic Donors
Two anonymous
COSMIC SEARCH, published by a non-profit scientific-educational organization, has been granted tax-exempt status by the Internal Revenue Service, so contributions are tax-deductible (but subscriptions are not). On a combination donation-subscription or donation-renewal, the amount over and above the magazine cost is tax deductible. For example, if a donor sends $30 (qualifying as a "Planetary Donor") and includes a two-year subscription at $18, the $12 difference is tax-deductible.
Donation categories are as follows:
Planetary: $30 per year
Stellar: $100 per year
Galactic: $500 per year
Cosmic: $1000 per year
Contributions, however, will be gratefully accepted in any amount. Checks should be made payable to Cosmic Quest, Inc., P.O. Box 293, Delaware, Ohio 43015.
Miscellaneous Quote
The following quote is not directly associated with any article.
Quote on page 18
Mankind's -Cide Side
We don't like a bug so we use a pesticide,
We don't like a plant so we use an herbicide,
Will these pesticides and herbicides get in the human food chain,
And cause mankind's inadvertent suicide?
Sedgewick Seti
Miscellaneous Photo
The following photo is not directly associated with any article.
Photo on page 20
1980 Index (Volume 2, Issues 5 - 8)
[This index was found on page 20 in the magazine.]
(Last number of listing is page number)
ABC's of Space, 5, 28; 6, 32; 7, 16; 8, 16
Articles
Bell, Trudy E., The Grand Analogy, 5, 2
Billingham, John, Forum: Billingham Interview, 5, 17
Brown, George H., Marconi, 6, 6
David, Leonard, Putting Our Best Signal Forward, 7, 2
Drake, Frank D., Biological Immortality, 7, 9; SETI, 8, 7
Dunwoody, Sharon, Scientific Writing, 8, 8
Fleury, Bruce E., Aliens in Our Oceans, 6, 2
Freudenthal, Hans, Towards a Cosmic Language, 5, 35
Gatewood, George, EPF Report, 8, 12
Good, I. J., The Chief Entities, 6, 13
Kardashev, Nikolai, Strategies of SETI, 7, 36; 8, 4
Kraus, John, Diana Waves Back, 7, 27; Radiation From Above, 5, 20
Lago, Don, In the Time Machine, 6, 30
Lovelock, James E., Alien Biospheres, 8, 2
Lurie, Joan, Science in the Mass Media, 7, 39
Pankonin, Vernon, The Radio Spectrum, 6, 40
Papagiannis, Michael, IAU Session, 5, 24
Smith, Marcia S., Confronting Political Realities, 7, 30
Stine, G. Harry, Space Industrialization, 6, 26
Tarter, Jill, Communication with ETI, 6, 18; Life in the Universe, 6, 19
Tinsley, Brian A., Long Term Limits to Growth, 8, 10
Trimble, Virginia, It's a Nice Planet to Visit, 5, 13
College Courses, 5, 46; 6, 38; 7, 40
Donors, 5, 47; 6, 25; 7, 48; 8, ii (inside front cover)
Editorials, 6, 10, 25; 8, 1
In Review (books)
Asimov, Isaac (film) 6, 17
Clarke, Arthur C., 7, 42
Goldsmith and Owen, 7, 42
Grey, Jerry, 5, 34
Hoffman, David (film), 8, 19
von Braun and Ordway, 7, 11
Off the Shelf Reviews
AIAA, 5, 48
Asimov, Isaac, 5, 48
Brown, Hanbury, 7, 35
Burnham, Jr., Robert, 7, 35
Clarke, Arthur C., 5, 48
Clarke, I.F., 5, 48
Cole, Dandridge, Coxe, 5, 48
Dose, Fox, et al, 5, 48
Dyson, Freeman, 5, 48
Edelson, E., 7, 35
Folsome, Clair E., 5, 48
French, A. P., 6, 12
Hallion and Crouch, 7, 35
Hapgood, Fred, 7, 35
Hardin, Garrett, 7, 35
Hartmann, William H., 6, 12
Heppenheimer, T. A., 5, 48
Hoffman, David, 8, 19
Jastrow, Robert, 7, 35
Kaufmann III, W. J., 6, 12
Man, John, 6, 12
National Academy of Science, 6, 12
Powers, Robert M., 6, 12
Sagan, Carl, 5, 48
Silk, Joseph, 6, 12
Stent, Gunther S., 6, 12
Stilley, Frank, 5, 48
Stine, G. Harry, 6, 12
Story, Ronald, 6, 12
Strickland, Glenn G., 7, 35
Sullivan, Walter, 5, 48
Tauber, G. E., 5, 48
Titulaer, Chriet, 6, 12
Wald, Robert M., 7, 35
Wells, Ernest H., 6, 12
Wunder, C. C., 7, 35
Young, Louise B., 7, 35
People, Places, Plans, 8, 13
SEnTInel, 5, 40; 6, 44; 7, 44; 8, 14
Space Happenings, 6, 11
|